Research Interests
Strong Correlations in Layered Materials
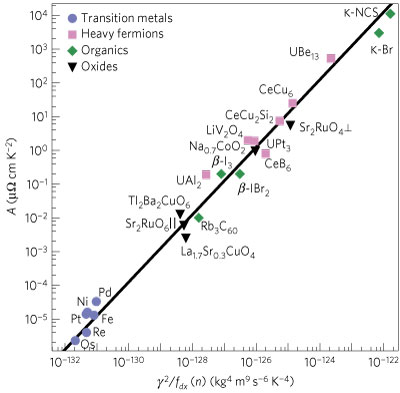
My research on strong correlations began with investigations of heavy fermions, transition metal oxides and layered organic charge transfer salts,
and the value of the Kadowaki-Woods ratio in these materials.
The Kadowaki-Woods ratio is the ratio of the electron-electron scattering contribution to resistivity to the square of the electronic contribution
to heat capacity. This ratio is constant for most heavy fermion materials, and for transition metals, taking different values in each. However,
layered materials do not have a constant value, and many exotic reasons have been posited, including proximity to a quantum critical point or a
strange temperature dependence of electron-phonon interactions in low dimensions. Some less exotic possibilities have also been discussed, for example
using volumetric (rather than molar) units in the heat capacity (to be consistent with the units of resistivity) reduces the variation in the KWR
in layered transition metal oxides.
If one of these arguments were correct, then the Kadowaki-Woods ratio would be a useful identifying feature of one of these unusual physical properties.
I, together with Ben Powell and John Fjærestad, investigated a simple phenomenological Fermi liquid theory. We found that:
- The KWR is not constant, in general.
- The reason that it appears constant in the heavy fermions and transition metals is due to other (material dependent) factors being constant.
- By correctly including these material-dependent factors one can formulate an new ratio which is universal in Fermi liquids.
These results are apparent in the above figure, taken from
our Nature Physics paper
(
arXiv here), showing experimental agreement over 10 decades of data for a wide range of materials.
I have used density functional theory to parameterise model Hamiltonians for low-dimensional strongly correlated materials such as the
Fabre salts and
Pd(dmit)2 derivatives. I applied exact diagonalisation and other many-body techniques to solve these model Hamitonials and study the interplay of frustration and anisotropy with correlations in low dimensions. In a
recent preprint, I showed that there are important qualitative differences between t-t' triangular lattices and fully anisotropic triangular lattices (FATL). This work was done in the context of Pd(dmit)2 materials, whose electronic structure is fairly well approximated by a t-t' model. However, we show that the relatively small deviation from t-t' seen in these materials is enough to greatly enhance the transition to the spin-liquid phase.
Photophysical Properties of Organometallic Complexes
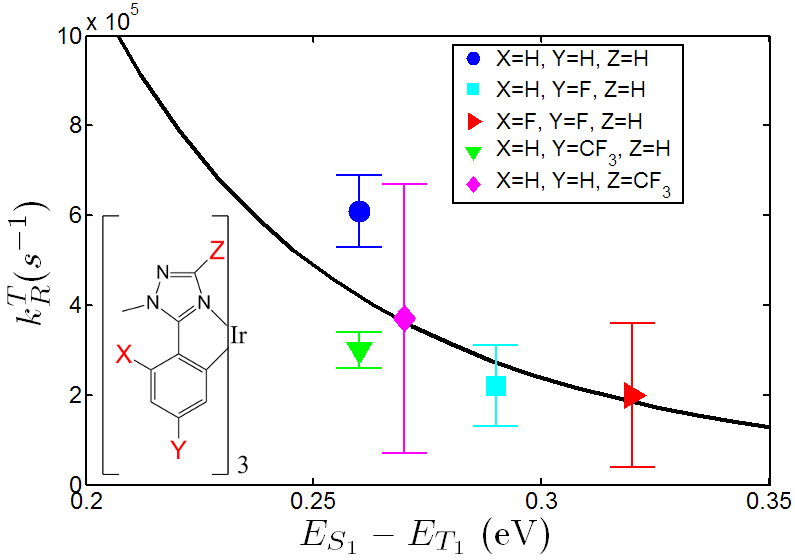
Organometallic complexes have potential applications as the optically active components of organic
light emitting diodes (OLEDs) and organic photovoltaics (OPV). Development of more effective
complexes may be aided by understanding their excited state properties. Some of the key theoretical challenges
in modelling these materials are determining the nature of the
emitting state, predicting the fraction of injected charges that form triplet excitations, and explaining
the sensitivity of device performance to small changes in the molecular structure of the organometallic complexes. For example, the
photo-luminescent quantum yield of an organometallic dye can vary by an order of magnitude as a result of a single chemical substitution of,
for example, hydrogen to fluorine.
Ross McKenzie, Ben Powell, and I have developed a semi-empirical effective Hamiltonian to investigate these properties. We found that
there is a single key parameter that strongly controls the singlet-triplet gap and the character of those states. As a result, small (0.1 eV)
changes in this parameter can cause the phosphorescent decay rate to vary by an order of magnitude. In a recent publication in
Chemical Physics Letters (
arXiv here) we showed that this
model can explain the correlation between the lowest excited singlet and triplet energy gap with the triplets radiative rate for a series of
closely related iridium cored blue phosphorescent complexes.
The initial work on this model is published in the
Journal of Chemical Physics (
arXiv here). We also wrote a review
of the various complementary approaches taken to modeling these kinds of systems, published in the
Journal of Materials Chemistry (
arXiv here).